النبات
مواضيع عامة في علم النبات
الجذور - السيقان - الأوراق
النباتات الوعائية واللاوعائية
البذور (مغطاة البذور - عاريات البذور)
الطحالب
النباتات الطبية
الحيوان
مواضيع عامة في علم الحيوان
علم التشريح
التنوع الإحيائي
البايلوجيا الخلوية
الأحياء المجهرية
البكتيريا
الفطريات
الطفيليات
الفايروسات
علم الأمراض
الاورام
الامراض الوراثية
الامراض المناعية
الامراض المدارية
اضطرابات الدورة الدموية
مواضيع عامة في علم الامراض
الحشرات
التقانة الإحيائية
مواضيع عامة في التقانة الإحيائية
التقنية الحيوية المكروبية
التقنية الحيوية والميكروبات
الفعاليات الحيوية
وراثة الاحياء المجهرية
تصنيف الاحياء المجهرية
الاحياء المجهرية في الطبيعة
أيض الاجهاد
التقنية الحيوية والبيئة
التقنية الحيوية والطب
التقنية الحيوية والزراعة
التقنية الحيوية والصناعة
التقنية الحيوية والطاقة
البحار والطحالب الصغيرة
عزل البروتين
هندسة الجينات
التقنية الحياتية النانوية
مفاهيم التقنية الحيوية النانوية
التراكيب النانوية والمجاهر المستخدمة في رؤيتها
تصنيع وتخليق المواد النانوية
تطبيقات التقنية النانوية والحيوية النانوية
الرقائق والمتحسسات الحيوية
المصفوفات المجهرية وحاسوب الدنا
اللقاحات
البيئة والتلوث
علم الأجنة
اعضاء التكاثر وتشكل الاعراس
الاخصاب
التشطر
العصيبة وتشكل الجسيدات
تشكل اللواحق الجنينية
تكون المعيدة وظهور الطبقات الجنينية
مقدمة لعلم الاجنة
الأحياء الجزيئي
مواضيع عامة في الاحياء الجزيئي
علم وظائف الأعضاء
الغدد
مواضيع عامة في الغدد
الغدد الصم و هرموناتها
الجسم تحت السريري
الغدة النخامية
الغدة الكظرية
الغدة التناسلية
الغدة الدرقية والجار الدرقية
الغدة البنكرياسية
الغدة الصنوبرية
مواضيع عامة في علم وظائف الاعضاء
الخلية الحيوانية
الجهاز العصبي
أعضاء الحس
الجهاز العضلي
السوائل الجسمية
الجهاز الدوري والليمف
الجهاز التنفسي
الجهاز الهضمي
الجهاز البولي
المضادات الحيوية
مواضيع عامة في المضادات الحيوية
مضادات البكتيريا
مضادات الفطريات
مضادات الطفيليات
مضادات الفايروسات
علم الخلية
الوراثة
الأحياء العامة
المناعة
التحليلات المرضية
الكيمياء الحيوية
مواضيع متنوعة أخرى
الانزيمات
Green Fluorescent Protein
المؤلف:
T. Misteli and D. L. Spector
المصدر:
Nature Biotech. 15, 961–964
الجزء والصفحة:
15-5-2016
2794
Green Fluorescent Protein (GFP(
GFP is a bioluminescent protein derived from the jellyfish Aequorea victoria. The gene was cloned in 1991, and the protein was found to have the unique property of emitting green light upon illumination with long-wave ultraviolet light without any requirement for additional cofactors or other substrates (1, 2). This has made its gene a most useful reporter gene. The 27-kDa GFP protein has a novel barrel-like protein structure comprised of 11 b-strands that surrounds and protects a cyclic tripeptide chromophore at its center (3). When excited by light at 470 nm, this chromophore fluoresces with an emission maxima of 509 nm that can be readily observed using conventional fluorescein isothiocyanate fluorescence optics.
The unique and robust properties of GFP have made it valuable for a wide range of applications. Most importantly, detection of light emission from GFP does not require any cell fixation or cell permeabilization, allowing for the first time visualization of a reporter gene in living cells. This feature has made GFP an exceptionally powerful tool in cell and transgenic biology, because expressing cells simply glow green under conventional fluorescence microscopy and confocal microscopy. Furthermore, GFP is observed in living cells, so dynamic events may be followed by making real-time or time-lapse observations. GFP has now been used as a reporter gene in cells from bacteria to human. Importantly, high levels of GFP expression have not been associated with any cellular toxicity; indeed, transgenic mice with ubiquitous expression of GFP thrive without problems. However, prolonged exposure of living cells with long-wave ultraviolet light might cause cell damage, and there may be nuclear damage caused by the generation of free radicals upon GFP excitation.
The b-barrel-like structure of GFP acts to protect its active site and has allowed numerous N-terminal and C-terminal fusion genes to be made and expressed, without loss of fluorescent activity. Consequently, GFP may be used to study the behavior of tagged proteins within living cells. Proteins have been tagged for a wide range of applications in different organisms. A few of the many examples include tagging ribonucleoprotein particles in Drosophila to study their trafficking into the developing egg chamber (4), tagging the histone H2B protein to study the dynamics of chromosome behavior in living human tumor cells (5), and studying cytoskeletal dynamics by binding GFP to F- actin in the slime mold Dictyostelium (6).
The second major use of GFP has been for cell-lineage analysis in developmental biology. GFP has not been found to be toxic in any cell type, and the fluorescence does not bleach when excited with 480-nm light. Furthermore, the protein is highly stable; for example, protein translated from messenger RNA microinjected into a Xenopus oocyte may still be detected at the feeding tadpole stage of development 5 days later. Thus the fate of the progeny of labeled cells can be followed over significant periods of time during development (7).
Following initial studies with the wild-type protein, several variant GFP isoforms have been generated by mutation analysis (2). Some of these mutations have improved the absorption and emission spectra of the protein, which increase the sensitivity and the versatility of the reporter gene. The mutation Ser65Thr results in an increased brightness of emission, faster fluorophore formation, and reduced photobleaching. Other novel isoforms are particularly valuable, because they emit light at new wavelengths; currently, blue-, red-, and cyan-shifted variants are available (Clontech; www.clontech.com), and these can be used in dual or multiple labeling studies with appropriate filters. While certain mutations affect GFP function, other ‘silent’ mutations have been generated that customize the gene for expression in mammalian cells or in bacteria by optimizing codon-usage preferences. Advances in GFP technology can be followed at the “Fluorescent protein Newsgroup” on the Internet (http://www.bio.net/hypermail/FLUORESCENT-PROTEINS).
The applications of GFP as a reporter gene are largely determined by its stability and sensitivity. The b-barrel-like structure that protects the chromophore is very stable, resisting temperatures up to 65°C and ionic detergents, and gives high resistance to proteinases (3). The long half-life of GFP makes it ideal for long-term cell-labeling or protein-tagging studies, but it is less suitable for monitoring dynamic changes in gene expression. To circumvent this problem, novel GFP fusion genes have been made with the PEST region of the mouse ornithine decarboxylase gene at the C-terminus, which destabilizes the GFP to protein degradation; it has a half-life of 1 to 2 hours (8).
Although GFP can be engineered to generate proteins of different half-lives and with different fluorogenic properties, less can be done to improve its sensitivity, because it lacks the ability to
amplify its signal through enzymatic turnover of added substrate. In all successful applications thus far, high levels of GFP expression have had to be obtained, achieving of the order of 106 molecules per cell or more. The sensitivity of detection is therefore orders of magnitude less than that of other enzyme-based reporter systems, and the detected expression is not tightly coupled to changes in gene transcription.
References
1. T. Misteli and D. L. Spector (1997) Nature Biotech. 15, 961–964.
2. A. B. Cubitt et al (1995) Trend Biochem. Sci. 20, 448–455.
3. G. N. Phillips Jr. (1997) Curr. Opin. Struct. Biol. 7, 821–827.
4. S. Wang and T. Hazelrigg (1994) Nature 369, 400–403.
5. T. Kanda, K. F. Sullivan, and G. M. Wahl (1998) Curr. Biol. 8, 377–385.
6. K. M. Pang, E. Lee, and D. A. Knecht (1998) Curr. Biol. 8, 405–408.
7. M. Zernicka-Goetz et al. (1996) Development 122, 3719–3724.
8. Clontechniques (1998) XIII (2), 16–17, Clontech Laboratories, Inc., Palo Alto, CA. (HYPERLINK http://www.clontech.com).
الاكثر قراءة في مواضيع عامة في الاحياء الجزيئي
اخر الاخبار
اخبار العتبة العباسية المقدسة
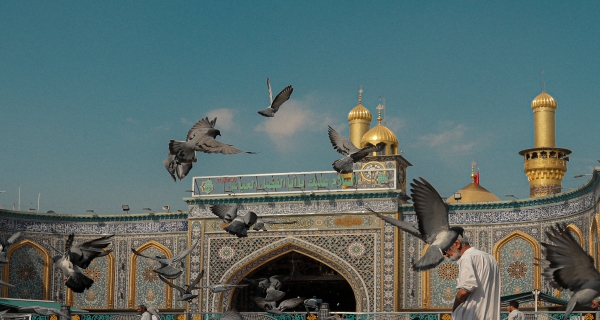
الآخبار الصحية
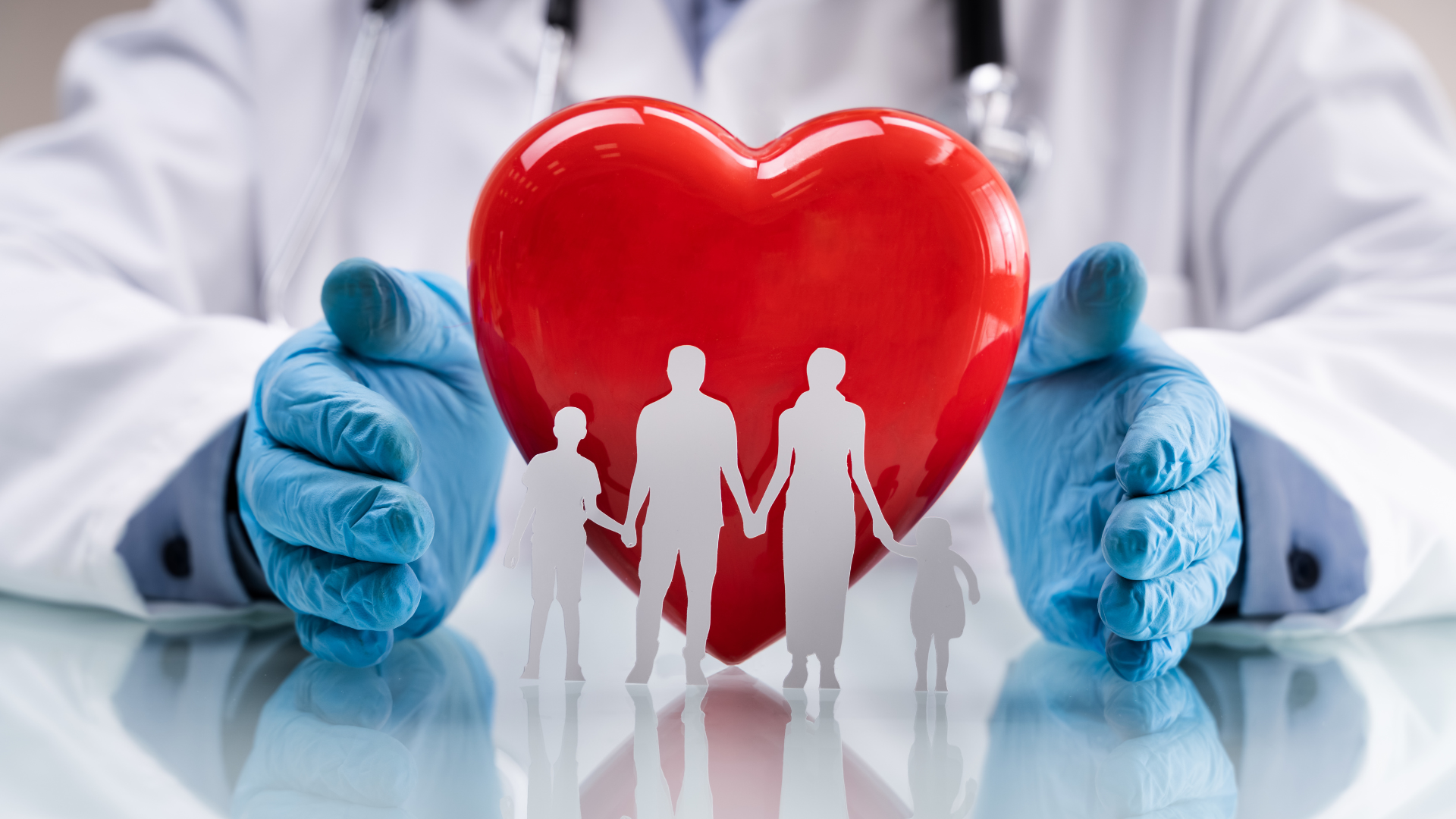