النبات
مواضيع عامة في علم النبات
الجذور - السيقان - الأوراق
النباتات الوعائية واللاوعائية
البذور (مغطاة البذور - عاريات البذور)
الطحالب
النباتات الطبية
الحيوان
مواضيع عامة في علم الحيوان
علم التشريح
التنوع الإحيائي
البايلوجيا الخلوية
الأحياء المجهرية
البكتيريا
الفطريات
الطفيليات
الفايروسات
علم الأمراض
الاورام
الامراض الوراثية
الامراض المناعية
الامراض المدارية
اضطرابات الدورة الدموية
مواضيع عامة في علم الامراض
الحشرات
التقانة الإحيائية
مواضيع عامة في التقانة الإحيائية
التقنية الحيوية المكروبية
التقنية الحيوية والميكروبات
الفعاليات الحيوية
وراثة الاحياء المجهرية
تصنيف الاحياء المجهرية
الاحياء المجهرية في الطبيعة
أيض الاجهاد
التقنية الحيوية والبيئة
التقنية الحيوية والطب
التقنية الحيوية والزراعة
التقنية الحيوية والصناعة
التقنية الحيوية والطاقة
البحار والطحالب الصغيرة
عزل البروتين
هندسة الجينات
التقنية الحياتية النانوية
مفاهيم التقنية الحيوية النانوية
التراكيب النانوية والمجاهر المستخدمة في رؤيتها
تصنيع وتخليق المواد النانوية
تطبيقات التقنية النانوية والحيوية النانوية
الرقائق والمتحسسات الحيوية
المصفوفات المجهرية وحاسوب الدنا
اللقاحات
البيئة والتلوث
علم الأجنة
اعضاء التكاثر وتشكل الاعراس
الاخصاب
التشطر
العصيبة وتشكل الجسيدات
تشكل اللواحق الجنينية
تكون المعيدة وظهور الطبقات الجنينية
مقدمة لعلم الاجنة
الأحياء الجزيئي
مواضيع عامة في الاحياء الجزيئي
علم وظائف الأعضاء
الغدد
مواضيع عامة في الغدد
الغدد الصم و هرموناتها
الجسم تحت السريري
الغدة النخامية
الغدة الكظرية
الغدة التناسلية
الغدة الدرقية والجار الدرقية
الغدة البنكرياسية
الغدة الصنوبرية
مواضيع عامة في علم وظائف الاعضاء
الخلية الحيوانية
الجهاز العصبي
أعضاء الحس
الجهاز العضلي
السوائل الجسمية
الجهاز الدوري والليمف
الجهاز التنفسي
الجهاز الهضمي
الجهاز البولي
المضادات الحيوية
مواضيع عامة في المضادات الحيوية
مضادات البكتيريا
مضادات الفطريات
مضادات الطفيليات
مضادات الفايروسات
علم الخلية
الوراثة
الأحياء العامة
المناعة
التحليلات المرضية
الكيمياء الحيوية
مواضيع متنوعة أخرى
الانزيمات
Calnexin/Calreticulin
المؤلف:
A. Helenius, E. S. Trombetta, D. N. Hebert, and J. S. Simons
المصدر:
Trends Cell Biol. 7, 193–200
الجزء والصفحة:
16-12-2015
2919
Calnexin/Calreticulin
Calnexin (also known as p88, IP90, and CATCHER) is a membrane-bound molecular chaperone present in the endoplasmic reticulum (ER) that binds selectively to many monoglucosylated glycoproteins that fold in this compartment during synthesis by ribosomes bound to the cytosolic face of the ER membrane. Calreticulin is a soluble homologue of calnexin, with the same glycan specificity, but occurs in the ER lumen. Together with other proteins, calnexin and calreticulin provide a means for improving the efficiency of protein folding and assembly in the ER compartment and form part of the quality-control system that ensures that only correctly folded and assembled proteins are transported from the ER compartment along the pathway followed by secretory proteins (1). Unlike some other molecular chaperones, calnexin and calreticulin are not stress response proteins, do not exhibit ATPase activity, and appear to recognize glucose residues in core glycans rather than hydrophobic surfaces in partially folded polypeptides (1). In some cell types, calreticulin also occurs in the nucleus and cytosol, where it binds to steroid hormone receptors and integrin molecules, respectively; these interactions may indicate roles for calreticulin in gene expression and cell signaling.
1. Discovery
An 88-kDa protein termed p88 was found in transient association with class I major histocompatibility molecules synthesized by several murine lymphoma cell lines (2). Newly synthesized-class I heavy chains bound rapidly to p88 before they associated with b2-microglobulin chains. In mutant cells that lack microglobulin, the incompletely assembled class I molecules exhibited prolonged interaction with p88 and were correspondingly impaired in their transport to the Golgi stacks (3). Moreover, microglobulin and peptides derived from the cytosol need to be assembled with the heavy chain before the latter can be released from p88. These findings led to the proposal that p88 is a molecular chaperone that promotes assembly of class I molecules by retaining intermediates in the ER until complete complexes are formed.
Independent pulse-chase experiments on a human ER protein termed IP90 showed that it transiently associates with many newly synthesized proteins, including the T-cell receptor (TCR), the B-cell antigen receptor, and class I molecules (4, 5). The binding of IP90 to TCR complexes lacking a chains was prolonged, consistent with a retention role for this protein in the ER for incompletely assembled complexes (6). Subsequently, p88 and IP90 were found to be identical with the Ca2+-binding phosphoprotein calnexin (4-7), a protein originally identified as one of several proteins labeled by phosphorylation of canine pancreatic microsomes with [g-32P]-GTP (8).
2. Structure
Calnexin is a type I nonglycosylated integral membrane protein of 573 amino acid residues, with a predicted molecular weight of 65,400 and with its substrate-binding domain in the lumen of the ER. It has a cytosolic tail of 89 residues that is phosphorylated and contains a C-terminal RKPRRE (Arg Lys Pro Arg Arg Glu) sequence that acts as an ER-retention signal (9). It also possesses four domains with high sequence similarity to calreticulin, the major calcium-binding protein of the ER lumen. Calreticulin contains 400 amino acid residues (46,000 molecular weight), which include both a KDEL ER-retrieval sequence and a strongly negatively charged region involved in Ca2+ binding at its C terminus (10). Both calnexin and calreticulin appear to function as monomers.
3. Function
Calnexin and calreticulin are both lectins that specifically recognize glycoproteins that contain monoglucosylated core glycans; they do not recognize glycans containing two, three or no glucose residues (11, 12). When nascent chains of glycoproteins enter the ER lumen, a core glycan containing three terminal glucose residues is added en bloc to specific asparagine sidechains. This addition of glycan may have evolved initially to make folding intermediates more soluble under the conditions of high protein concentration that characterize the ER lumen. These glucose residues are then rapidly removed one at a time by glucosidases I and II in the ER lumen. Another lumenal enzyme called UDP-Glc:glycoprotein glucosyltransferase then adds back one glucose residue. Thus glycans containing single terminal glucose residues can arise either as intermediates in the glucose-trimming process or after regeneration by the transferase. Such monoglucosylated glycans then bind to calnexin and calreticulin. In the case of pancreatic ribonuclease B, this binding occurs solely through the glycan and does not involve additional recognition of the protein moiety by the lectins (13, 14), but such additional recognition may occur in the case of other proteins (11, 15).
The function of the binding of calnexin and calreticulin to monoglucosylated glycoproteins appears to be to assist their correct folding by preventing aggregation of partially folded or misfolded chains and ensuring retention of the latter until they are degraded. Evidence for this conclusion comes from experiments in which proteins unable to fold correctly because of mutation remain bound to these chaperones for much longer than normal proteins and are eventually degraded (16-18). Additional possible roles include the suppression of formation of nonnative disulfide bonds (19) and the premature assembly of oligomers (18).
The distinction between correctly folded and partially folded chains is not made by these lectins but by the glucosyltransferase. This enzyme has the unusual property of distinguishing, by an unknown mechanism, between subtly different protein conformations; it adds glucose to the core glycan only if the protein attached to this glycan is not correctly folded (20, 21). Such reglucosylated glycoproteins will then bind back to calnexin and calreticulin. The removal and addition of the terminal glucose residue then continues until the protein has folded sufficiently to be no longer recognized by the transferase. The binding of calnexin to ribonuclease B in vitro is dynamic, indicating that the binding is readily reversible; glucosidase II cannot remove terminal glucose residues while calnexin is bound to the glycoprotein (14).
Calnexin and calreticulin associate with a wide range of proteins in the ER, including soluble secretory proteins, extracellular matrix molecules, ion channels, membrane receptors, and various glycoproteins of viruses. They also retain misfolded proteins that accumulate in some ER-storage diseases such as cystic fibrosis and a1-antitrypsin deficiency (22). Addition of glucosidase inhibitors to cells results in reduced rates of secretion of many glycoproteins, presumably due to the inhibition of their binding to the chaperones (23). All these observations support the view that the functions of calnexin and calreticulin are important for cell function. Nevertheless, viable mammalian cell lines are known that lack either glucosidase I or II or calnexin, while mutants of Saccharomyces
cerevisiae that lack genes for any one of these three proteins show no growth defect. However, mutants of Saccharomyces pombe that lack calnexin are not viable. Both species of Saccharomyces appear to lack calreticulin. These discrepancies may indicate some redundancy and overlap of function amongst the chaperones in the ER compartment, which include BiP, hsp90, and protein disulfide isomerase, as well as calnexin and calreticulin (1).
References
1. A. Helenius, E. S. Trombetta, D. N. Hebert, and J. S. Simons (l997) Trends Cell Biol. 7, 193–200.
2. E. Degen and D. B. Williams (1991) J. Cell Biol. 112, 1099–1115.
3. E. Degen, M. F. Cohen-Doyle, and D. B. Williams (1992) J. Exp. Med. 175, 1653–1661.
4. F. Hochenstenbach, V. David, S. Watkins, and M. B. Brenner (1992) Proc. Natl. Acad. Sci. USA 89, 4734–4738.
5. K. Galvin et al. (1992) Proc. Natl. Acad. Sci USA 89, 8452–8456.
6. V. David, F. Hochstenbach, S. Rajagopalan, and M. B. Brenner (1993) J. Biol. Chem. 2689585-9592 , .
7. N. Ahluwalia, J. J. M. Bergeron, I. Wada, E. Degen, and D. B. Williams (1992) J. Biol. Chem. 267, 10914-10918 .
8. I. Wada, D. Rindress, P. H. Cameron, W.-J. Ou, J. J. Dohery, D. Louvard, A. W. Bell, D.
Dignard, D. Y. Thomas, and J. J. M. Bergeron (1991) J. Biol. Chem. 266, 19599–19610.
9. M. Michalak, R. E. Milner, K. Burns, and M. Opas (1992) Biochem. J. 285, 681–692.
10. B. Sonnichsen, J. Fullefrug, P. Nguyen, W. Diekmann, D. G. Robinson, and G. Mieskes (1994(J. Cell Sci. 107, 2705–2717.
11. F. E. Ware, A. Vassilakos, P. A. Petersen, M. R. Jackson, M. A. Lehrmann, and D. B. Williams (1995) J. Biol. Chem. 270, 4697–4704.
12. R. G. Spiro, Q. Zhu, V. Bhoyroo, and H.-D. Soling (1996) J. Biol. Chem. 271, 11588–11594.
13. A. R. Rodan, J. F. Simons, E. S. Trombetta, and A. Helenius (1996) EMBO J. 15, 6921–6930.
14. A. Zapun, S. M. Petrescu, P. M. Rudd, A. R. Dwek, D. Y. Thomas, and J. J. M. Bergeron (1997(Cell 88, 29–38.
15. D. B. Williams (1995) Biochem. Cell Biol. 73, 123–132.
16. C. Hammond and A. Helenius (1994) Science 266, 456–458.
17. D. F. Qu, J. H. Teckman, S. Omura, and D. H. Perlmutter (1996) J. Biol. Chem. 271, 22791–22795.
18.D. N. Hebert, B. Foellmer, and A. Helenius (1996) EMBO J. 15, 2961–2968.
19. W. Chen, J. Helenius, I. Braakman, and A. Helenius (1995) Proc. Natl. Acad. Sci. USA 92, 6229-6233 .
20. M. C. Sousa, M. A. Ferrero-Garcia, and A. J. Parodi (1992) Biochemistry 31, 97–105.
21. S. E. Trombetta and A. J. Parodi (1992) J. Biol. Chem. 267, 9236–9240.
22. P. J. Thomas, B. Qu, and P. L. Pederson (1995) Trends Biochem. Sci. 20, 456–459.
23. V. Gross, T. Andus, T. A. Tranhl, R. T. Schwartz, K. Decker, and P. C. Heinrich (1983) J. Biol. Chem. 258, 12203–12209.
الاكثر قراءة في مواضيع عامة في الاحياء الجزيئي
اخر الاخبار
اخبار العتبة العباسية المقدسة
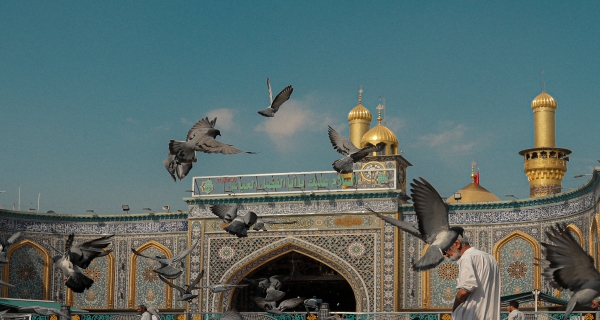
الآخبار الصحية
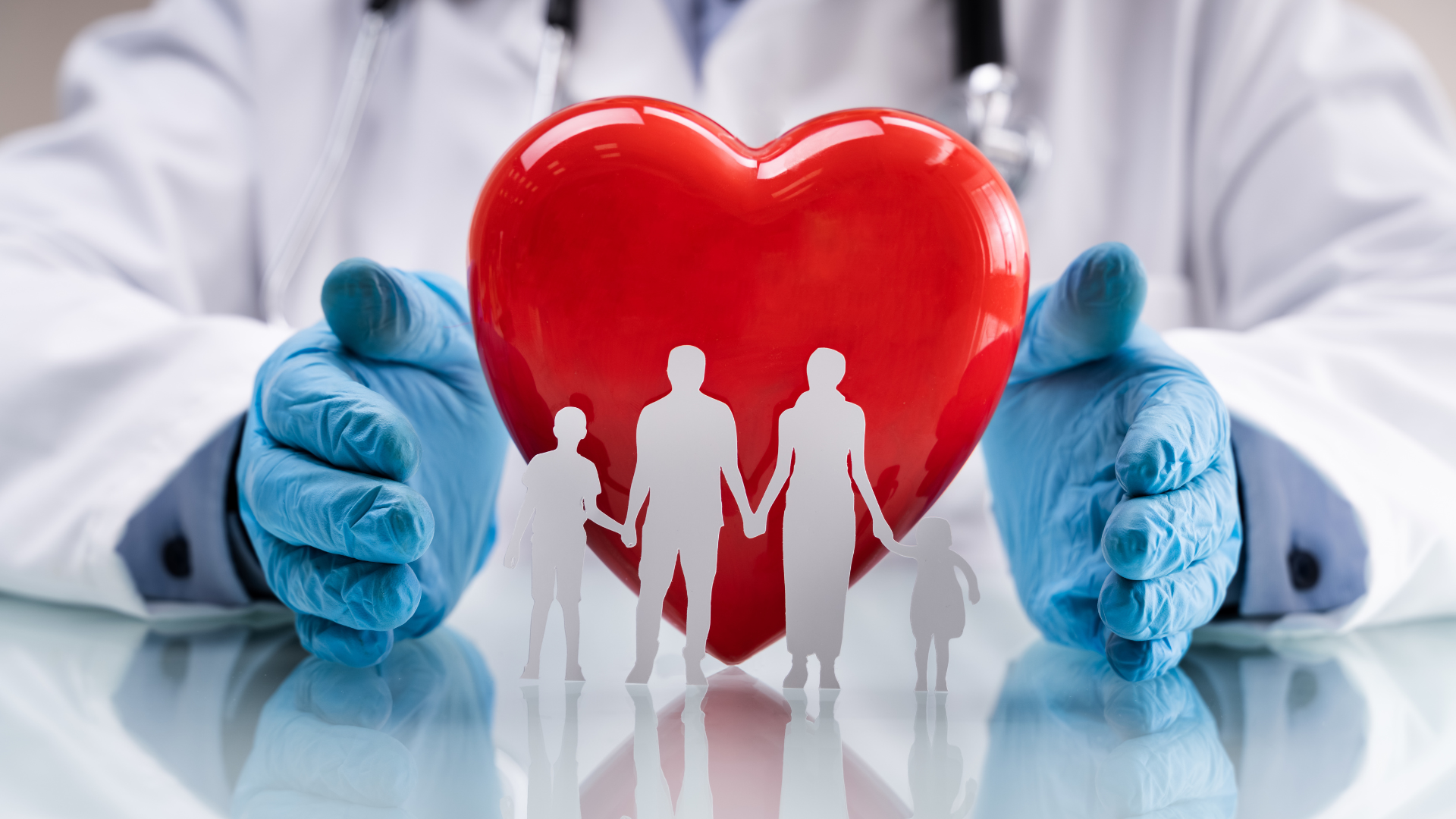