تاريخ الفيزياء
علماء الفيزياء
الفيزياء الكلاسيكية
الميكانيك
الديناميكا الحرارية
الكهربائية والمغناطيسية
الكهربائية
المغناطيسية
الكهرومغناطيسية
علم البصريات
تاريخ علم البصريات
الضوء
مواضيع عامة في علم البصريات
الصوت
الفيزياء الحديثة
النظرية النسبية
النظرية النسبية الخاصة
النظرية النسبية العامة
مواضيع عامة في النظرية النسبية
ميكانيكا الكم
الفيزياء الذرية
الفيزياء الجزيئية
الفيزياء النووية
مواضيع عامة في الفيزياء النووية
النشاط الاشعاعي
فيزياء الحالة الصلبة
الموصلات
أشباه الموصلات
العوازل
مواضيع عامة في الفيزياء الصلبة
فيزياء الجوامد
الليزر
أنواع الليزر
بعض تطبيقات الليزر
مواضيع عامة في الليزر
علم الفلك
تاريخ وعلماء علم الفلك
الثقوب السوداء
المجموعة الشمسية
الشمس
كوكب عطارد
كوكب الزهرة
كوكب الأرض
كوكب المريخ
كوكب المشتري
كوكب زحل
كوكب أورانوس
كوكب نبتون
كوكب بلوتو
القمر
كواكب ومواضيع اخرى
مواضيع عامة في علم الفلك
النجوم
البلازما
الألكترونيات
خواص المادة
الطاقة البديلة
الطاقة الشمسية
مواضيع عامة في الطاقة البديلة
المد والجزر
فيزياء الجسيمات
الفيزياء والعلوم الأخرى
الفيزياء الكيميائية
الفيزياء الرياضية
الفيزياء الحيوية
الفيزياء العامة
مواضيع عامة في الفيزياء
تجارب فيزيائية
مصطلحات وتعاريف فيزيائية
وحدات القياس الفيزيائية
طرائف الفيزياء
مواضيع اخرى
Approximating irrational numbers
المؤلف:
Richard Feynman, Robert Leighton and Matthew Sands
المصدر:
The Feynman Lectures on Physics
الجزء والصفحة:
Volume I, Chapter 22
2024-03-10
1418
The next problem comes with what happens with the irrational powers. Suppose that we want to define, for instance, 10√2. In principle, the answer is simple enough. If we approximate the square root of 2 to a certain number of decimal places, then the power is rational, and we can take the approximate root, using the above method, and get an approximation to 10√2. Then we may run it up a few more decimal places (it is again rational), take the appropriate root, this time a much higher root because there is a much bigger denominator in the fraction, and get a better approximation. Of course, we are going to get some enormously high roots involved here, and the work is quite difficult. How can we cope with this problem?
In the computations of square roots, cube roots, and other small roots, there is an arithmetical process available by which we can get one decimal place after another. But the amount of labor needed to calculate irrational powers and the logarithms that go with them (the inverse problem) is so great that there is no simple arithmetical process we can use. Therefore, tables have been built up which permit us to calculate these powers, and these are called the tables of logarithms, or the tables of powers, depending on which way the table is set up. It is merely a question of saving time; if we must raise some number to an irrational power, we can look it up rather than having to compute it. Of course, such a computation is just a technical problem, but it is an interesting one, and of great historical value. In the first place, not only do we have the problem of solving x=10√2, but we also have the problem of solving 10x=2, or x=log10 2. This is not a problem where we have to define a new kind of number for the result, it is merely a computational problem. The answer is simply an irrational number, an unending decimal, not a new kind of a number.
Let us now discuss the problem of calculating solutions of such equations. The general idea is really very simple. If we could calculate 101, and 104/10, and 101/100, and 104/1000 and so on, and multiply them all together, we would get 101.414… or 10√2, and that is the general idea on which things work. But instead of calculating 101/10 and so on, we shall calculate 101/2, 101/4, and so on. Before we start, we should explain why we make so much work with 10, instead of some other number. Of course, we realize that logarithm tables are of great practical utility, quite aside from the mathematical problem of taking roots, since with any base at all,
We are all familiar with the fact that one can use this fact in a practical way to multiply numbers if we have a table of logarithms. The only question is, with what base b shall we compute? It makes no difference what base is used; we can use the same principle all the time, and if we are using logarithms to any particular base, we can find logarithms to any other base merely by a change in scale, a multiplying factor. If we multiply Eq. (22.3) by 61, it is just as true, and if we had a table of logs with a base b, and somebody else multiplied all of our table by 61, there would be no essential difference. Suppose that we know the logarithms of all the numbers to the base b. In other words, we can solve the equation ba=c for any c because we have a table. The problem is to find the logarithm of the same number c to some other base, let us say the base x. We would like to solve xa′=c. It is easy to do, because we can always write x=bt, which defines t, knowing x and b. As a matter of fact, t=logb x. Then if we put that in and solve for a′, we see that (bt)a′=ba′t=c. In other words, ta′ is the logarithm of c in base b. Thus a′=a/t. Thus, logs to base x are just 1/t, which is a constant, times the logs to the base, b. Therefore, any log table is equivalent to any other log table if we multiply by a constant, and the constant is 1/logb x. This permits us to choose a particular base, and for convenience we take the base 10. (The question may arise as to whether there is any natural base, any base in which things are somehow simpler, and we shall try to find an answer to that later. At the moment we shall just use the base 10.)
Table 22–1
Successive Square Roots of Ten
Now let us see how to calculate logarithms. We begin by computing successive square roots of 10, by cut and try. The results are shown in Table 22–1. The powers of 10 are given in the first column, and the result, 10s, is given in the third column. Thus 101=10. The one-half power of 10 we can easily work out, because that is the square root of 10, and there is a known, simple process for taking square roots of any number.1 Using this process, we find the first square root to be 3.16228. What good is that? It already tells us something, it tells us how to take 100.5, so we now know at least one logarithm, if we happen to need the logarithm of 3.16228, we know the answer is close to 0.50000. But we must do a little bit better than that; we clearly need more information. So we take the square root again, and find 101/4, which is 1.77828. Now we have the logarithm of more numbers than we had before, 1.250 is the logarithm of 17.78 and, incidentally, if it happens that somebody asks for 100.75, we can get it, because that is 10(0.5+0.25); it is therefore the product of the second and third numbers. If we can get enough numbers in column s to be able to make up almost any number, then by multiplying the proper things in column 3, we can get 10 to any power; that is the plan. So we evaluate ten successive square roots of 10, and that is the main work which is involved in the calculations.
Why don’t we keep on going for more and more accuracy? Because we begin to notice something. When we raise 10 to a very small power, we get 1 plus a small amount. The reason for this is clear, because we are going to have to take the 1000th power of 101/1000 to get back to 10, so we had better not start with too big a number; it has to be close to 1. What we notice is that the small numbers that are added to 1 begin to look as though we are merely dividing by 2 each time; we see 1815 becomes 903, then 450, 225; so it is clear that, to an excellent approximation, if we take another root, we shall get 1.00112 something, and rather than actually take all the square roots, we guess at the ultimate limit. When we take a small fraction Δ/1024 as Δ approaches zero, what will the answer be? Of course, it will be some number close to 1+0.0022511Δ. Not exactly 1+0.0022511Δ, however—we can get a better value by the following trick: we subtract the 1, and then divide by the power s. This ought to correct all the excesses to the same value. We see that they are very closely equal. At the top of the table, they are not equal, but as they come down, they get closer and closer to a constant value. What is the value? Again, we look to see how the series is going, how it has changed with s. It changed by 211, by 104, by 53, by 26. These changes are obviously half of each other, very closely, as we go down. Therefore, if we kept going, the changes would be 13, 7, 3, 2 and 1, more or less, or a total of 26. Thus, we have only 26 more to go, and so we find that the true number is 2.3025. (Actually, we shall later see that the exact number should be 2.3026, but to keep it realistic, we shall not alter anything in the arithmetic.) From this table we can now calculate any power of 10, by compounding the power out of 1024ths.
Let us now actually calculate a logarithm, because the process we shall use is where logarithm tables actually come from. The procedure is shown in Table 22–2, and the numerical values are shown in Table 22–1 (columns 2 and 3).
Table 22–2
Calculation of a logarithm: log10 2
Suppose we want the logarithm of 2. That is, we want to know to what power we must raise 10 to get 2. Can we raise 10 to the 1/2 power? No; that is too big. In other words, we can see that the answer is going to be bigger than 1/4, and less than 1/2. Let us take the factor 101/4 out; we divide 2 by 1.778…, and get 1.124…, and so on, and now we know that we have taken away 0.250000 from the logarithm. The number 1.124…, is now the number whose logarithm we need. When we are finished, we shall add back the 1/4, or 256/1024. Now we look in the table for the next number just below 1.124…, and that is 1.074607. We therefore divide by 1.074607 and get 1.046598. From that we discover that 2 can be made up of a product of numbers that are in Table 22–1, as follows:
There was one factor (1.000573) left over, naturally, which is beyond the range of our table. To get the logarithm of this factor, we use our result that 10Δ/1024≈1+2.3025Δ/1024. We find Δ=0.254. Therefore, our answer is 10 to the following power: (256+32+16+4+0.254)/1024. Adding those together, we get 308.254/1024. Dividing, we get 0.30103, so we know that the log10 2=0.30103, which happens to be right to 5 figures!
This is how logarithms were originally computed by Mr. Briggs of Halifax, in 1620. He said, “I computed successively 54 square roots of 10.” We know he really computed only the first 27, because the rest of them can be obtained by this trick with Δ. His work involved calculating the square root of 10 twenty-seven times, which is not much more than the ten times we did; however, it was more work because he calculated to sixteen decimal places, and then reduced his answer to fourteen when he published it, so that there were no rounding errors. He made tables of logarithms to fourteen decimal places by this method, which is quite tedious. But all logarithm tables for three hundred years were borrowed from Mr. Briggs’ tables by reducing the number of decimal places. Only in modern times, with the WPA and computing machines, have new tables been independently computed. There are much more efficient methods of computing logarithms today, using certain series expansions.
In the above process, we discovered something rather interesting, and that is that for very small powers ϵ we can calculate 10ϵ easily; we have discovered that 10ϵ=1+2.3025ϵ, by sheer numerical analysis. Of course, this also means that 10n/2.3025=1+n if n is very small. Now logarithms to any other base are merely multiples of logarithms to the base 10. The base 10 was used only because we have 10 fingers, and the arithmetic of it is easy, but if we ask for a mathematically natural base, one that has nothing to do with the number of fingers on human beings, we might try to change our scale of logarithms in some convenient and natural manner, and the method which people have chosen is to redefine the logarithms by multiplying all the logarithms to the base 10 by 2.3025… This then corresponds to using some other base, and this is called the natural base, or base e. Note that loge (1+n)≈n, or en≈1+n as n→0.
It is easy enough to find out what e is: e=101/2.3025… or 100.434310…, an irrational power. Our table of the successive square roots of 10 can be used to compute, not just logarithms, but also 10 to any power, so let us use it to calculate this natural base e. For convenience we transform 0.434310… into 444.73/1024. Now, 444.73 is 256+128+32+16+8+4+0.73. Therefore e, since it is an exponent of a sum, will be a product of the numbers
(The only problem is the last one, which is 0.73, and which is not in the table, but we know that if Δ is small enough, the answer is 1+0.0022486Δ.) When we multiply all these together, we get 2.7184 (it should be 2.7183, but it is good enough). The use of such tables, then, is the way in which irrational powers and the logarithms of irrational numbers are all calculated. That takes care of the irrationals.
_____________________________________________________________________
Margin
1- There is a definite arithmetic procedure, but the easiest way to find the square root of any number N is to choose some a fairly close, find N/a, average a′=1/2 [a+(N/a)], and use this average a′ for the next choice for a. The convergence is very rapid—the number of significant figures doubles each time.
الاكثر قراءة في الفيزياء الرياضية
اخر الاخبار
اخبار العتبة العباسية المقدسة
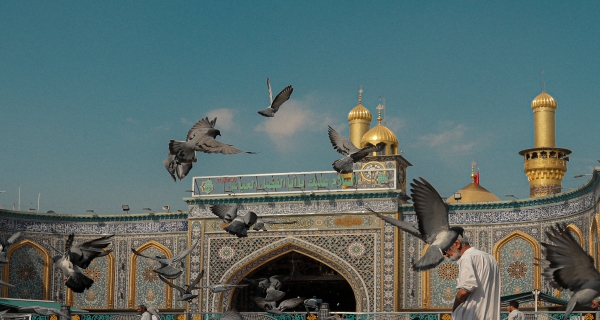
الآخبار الصحية
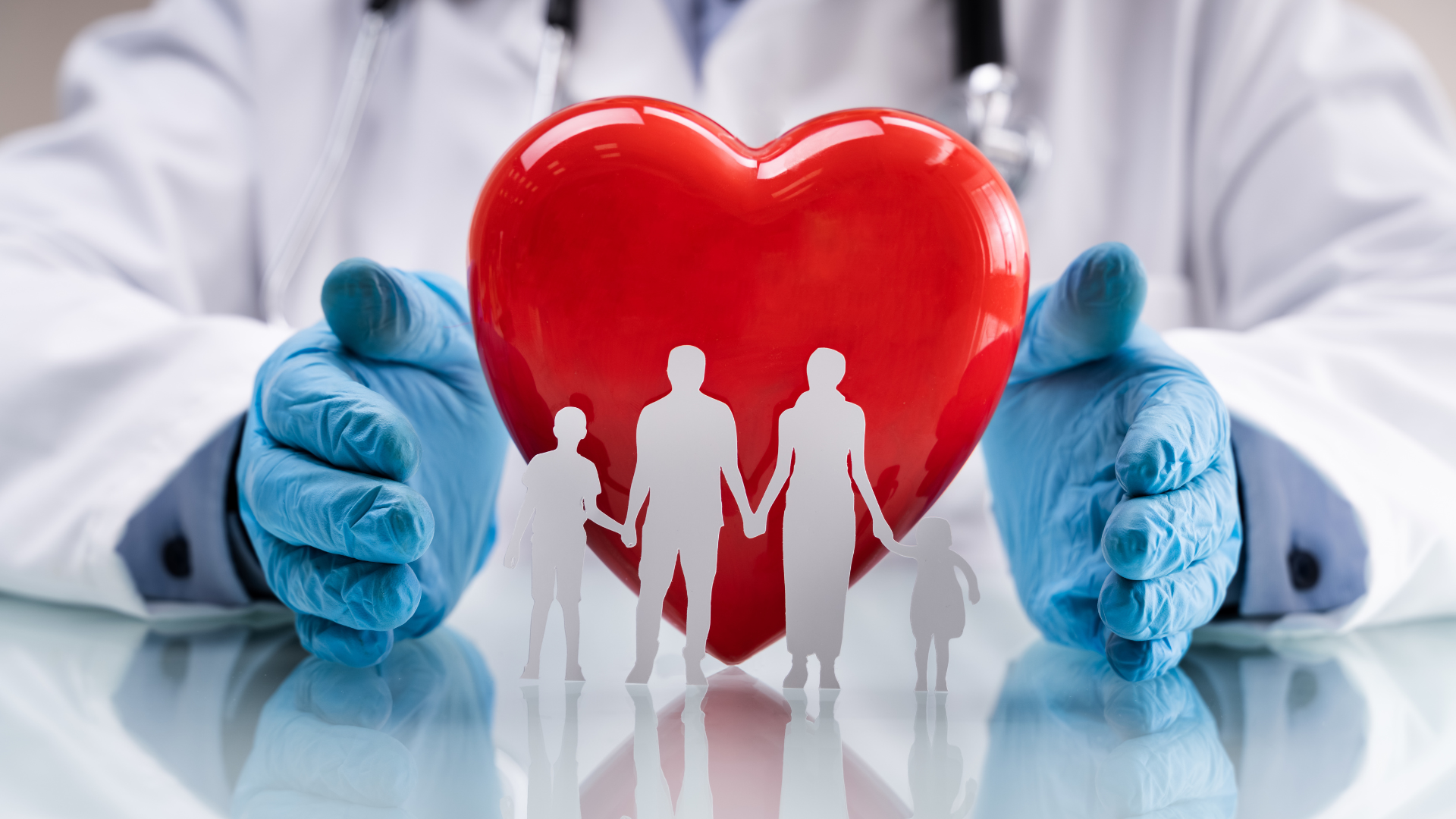