النبات
مواضيع عامة في علم النبات
الجذور - السيقان - الأوراق
النباتات الوعائية واللاوعائية
البذور (مغطاة البذور - عاريات البذور)
الطحالب
النباتات الطبية
الحيوان
مواضيع عامة في علم الحيوان
علم التشريح
التنوع الإحيائي
البايلوجيا الخلوية
الأحياء المجهرية
البكتيريا
الفطريات
الطفيليات
الفايروسات
علم الأمراض
الاورام
الامراض الوراثية
الامراض المناعية
الامراض المدارية
اضطرابات الدورة الدموية
مواضيع عامة في علم الامراض
الحشرات
التقانة الإحيائية
مواضيع عامة في التقانة الإحيائية
التقنية الحيوية المكروبية
التقنية الحيوية والميكروبات
الفعاليات الحيوية
وراثة الاحياء المجهرية
تصنيف الاحياء المجهرية
الاحياء المجهرية في الطبيعة
أيض الاجهاد
التقنية الحيوية والبيئة
التقنية الحيوية والطب
التقنية الحيوية والزراعة
التقنية الحيوية والصناعة
التقنية الحيوية والطاقة
البحار والطحالب الصغيرة
عزل البروتين
هندسة الجينات
التقنية الحياتية النانوية
مفاهيم التقنية الحيوية النانوية
التراكيب النانوية والمجاهر المستخدمة في رؤيتها
تصنيع وتخليق المواد النانوية
تطبيقات التقنية النانوية والحيوية النانوية
الرقائق والمتحسسات الحيوية
المصفوفات المجهرية وحاسوب الدنا
اللقاحات
البيئة والتلوث
علم الأجنة
اعضاء التكاثر وتشكل الاعراس
الاخصاب
التشطر
العصيبة وتشكل الجسيدات
تشكل اللواحق الجنينية
تكون المعيدة وظهور الطبقات الجنينية
مقدمة لعلم الاجنة
الأحياء الجزيئي
مواضيع عامة في الاحياء الجزيئي
علم وظائف الأعضاء
الغدد
مواضيع عامة في الغدد
الغدد الصم و هرموناتها
الجسم تحت السريري
الغدة النخامية
الغدة الكظرية
الغدة التناسلية
الغدة الدرقية والجار الدرقية
الغدة البنكرياسية
الغدة الصنوبرية
مواضيع عامة في علم وظائف الاعضاء
الخلية الحيوانية
الجهاز العصبي
أعضاء الحس
الجهاز العضلي
السوائل الجسمية
الجهاز الدوري والليمف
الجهاز التنفسي
الجهاز الهضمي
الجهاز البولي
المضادات الحيوية
مواضيع عامة في المضادات الحيوية
مضادات البكتيريا
مضادات الفطريات
مضادات الطفيليات
مضادات الفايروسات
علم الخلية
الوراثة
الأحياء العامة
المناعة
التحليلات المرضية
الكيمياء الحيوية
مواضيع متنوعة أخرى
الانزيمات
The Bacterial Genome Is Supercoiled and Has Four Macrodomains
المؤلف:
JOCELYN E. KREBS, ELLIOTT S. GOLDSTEIN and STEPHEN T. KILPATRICK
المصدر:
LEWIN’S GENES XII
الجزء والصفحة:
21-3-2021
2135
The Bacterial Genome Is Supercoiled and Has Four Macrodomains
KEY CONCEPTS
-The nucleoid has about 400 independent negatively supercoiled domains.
-The average density of supercoiling is approximately 1 turn/100 bp.
- The circular bacterial genome has four macrodomains (ori, right, ter, left) that adopt replication-associated spatial patterns.
The DNA of the bacterial nucleoid isolated in vitro behaves as a closed duplex structure, as judged by its response to ethidium bromide. This small molecule intercalates between base pairs to generate positive superhelical turns in “closed” circular DNA molecules; that is, molecules in which both strands have covalent integrity. (In “open” circular molecules, which contain a nick in one strand, or with linear molecules, the DNA can rotate freely in response to the intercalation, thus relieving the tension.)
In a natural closed DNA that is negatively supercoiled, the intercalation of ethidium bromide first removes the negative supercoils and then introduces positive supercoils. The amount of ethidium bromide needed to achieve zero supercoiling is a measure of the original density of negative supercoils.
Some nicks occur in the compact nucleoid during its isolation; they can also be generated by limited treatment with DNase. This does not, however, abolish the ability of ethidium bromide to introduce positive supercoils. This capacity of the genome to retain its response to ethidium bromide in the face of nicking reflects the existence of many independent chromosomal domains, and that the supercoiling in each domain is not affected by events in the other domains.
Early data suggested that each domain consists of around 40 kilobases (kb) of DNA, but more recent analysis suggests that the domains can be smaller, about 10 kb each. This would correspond to approximately 400 domains in the E. coli genome. It is likely that there is in fact a range of domain sizes. The ends of the domains appear to be randomly distributed instead of located at predetermined sites on the chromosome.
The existence of separate domains could permit different degrees of supercoiling to be maintained in different regions of the genome.
This could be relevant in considering the different susceptibilities of particular bacterial promoters to supercoiling .
Supercoiling in the genome can in principle take either of two forms:
- If a supercoiled DNA is free, its path is unconstrained, and negative supercoils generate a state of torsional tension that is transmitted freely along the DNA within a domain. Torsional tension resulting from negative supercoils can be relieved by unwinding the double helix, as described in the chapter titled Genes Are DNA and Encode RNAs and Polypeptides. The DNA
is in a dynamic equilibrium between the states of tension and unwinding.
- Supercoiling can be constrained if proteins are bound to the DNA to hold it in a particular three-dimensional configuration. In this case, the supercoils are represented by the path the DNA follows in its fixed association with the proteins. The energy of interaction between the proteins and the supercoiled DNA stabilizes the nucleic acid so that no tension is transmitted along the molecule.
Measurements of supercoiling in vitro encounter the difficulty that constraining proteins might have been lost during isolation.
However, various approaches suggest that DNA is under torsional stress in vivo. One approach is to measure the effect of nicking the DNA.
Unconstrained supercoils are released by nicking, whereas constrained supercoils are unaffected. Nicking releases about 50% of the overall supercoiling. This suggests that about half of the supercoiling is transmitted as tension along DNA, with the other half being absorbed by protein binding. Another approach uses the crosslinking reagent psoralen, which binds more readily to DNA when it is under torsional tension. The reaction of psoralen with E. coli DNA in vivo corresponds to an average density of 1 negative superhelical turn/200 bp (σ = −0.05).
We also can examine the ability of cells to form alternative DNA structures; for example, to generate cruciforms (intrastrand base pairing) at palindromic sequences. From the change in linking number that is required to drive such reactions, it is possible to calculate the original supercoiling density. This approach suggests an average density of σ = −0.025, or 1 negative superhelical turn/100 bp.
Thus supercoils do appear to create torsional tension in vivo. There might be variation about an average level, and the precise range of densities is difficult to measure. It is, however, clear that
the level is sufficient to exert significant effects on DNA structure—for example, in assisting melting in particular regions such as origins or promoters.
Operating at a larger scale, nucleoid structural features, including macrodomains, have recently been observed using genetic and live imaging techniques.
FIGURE 1 Large-scale organizational patterns of the macrodomains in bacteria. The domains are delimited by the origin (ori) and termination (ter) regions, creating two different replichores
termed left and right.
FIGURE .1 shows two large-scale organizational patterns that have been observed in bacteria. The domains are delimited by the origin (ori) and termination (ter) regions, creating two different replichores termed left and right. The two patterns, referred to as ori-ter and left-ori-right, have been observed to be prevalent in different species of bacteria. Interestingly, they have both been shown to occur in Bacillus subtilis, but at different times during cell cycle progression. In this regard, bacterial and eukaryotic genomes display a similar phenomenon in which genome structure and dynamics is linked to progression through the cell cycle and DNA
synthesis phases.
الاكثر قراءة في مواضيع عامة في الاحياء الجزيئي
اخر الاخبار
اخبار العتبة العباسية المقدسة
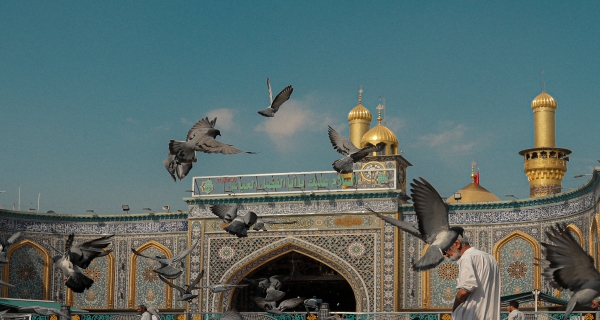
الآخبار الصحية
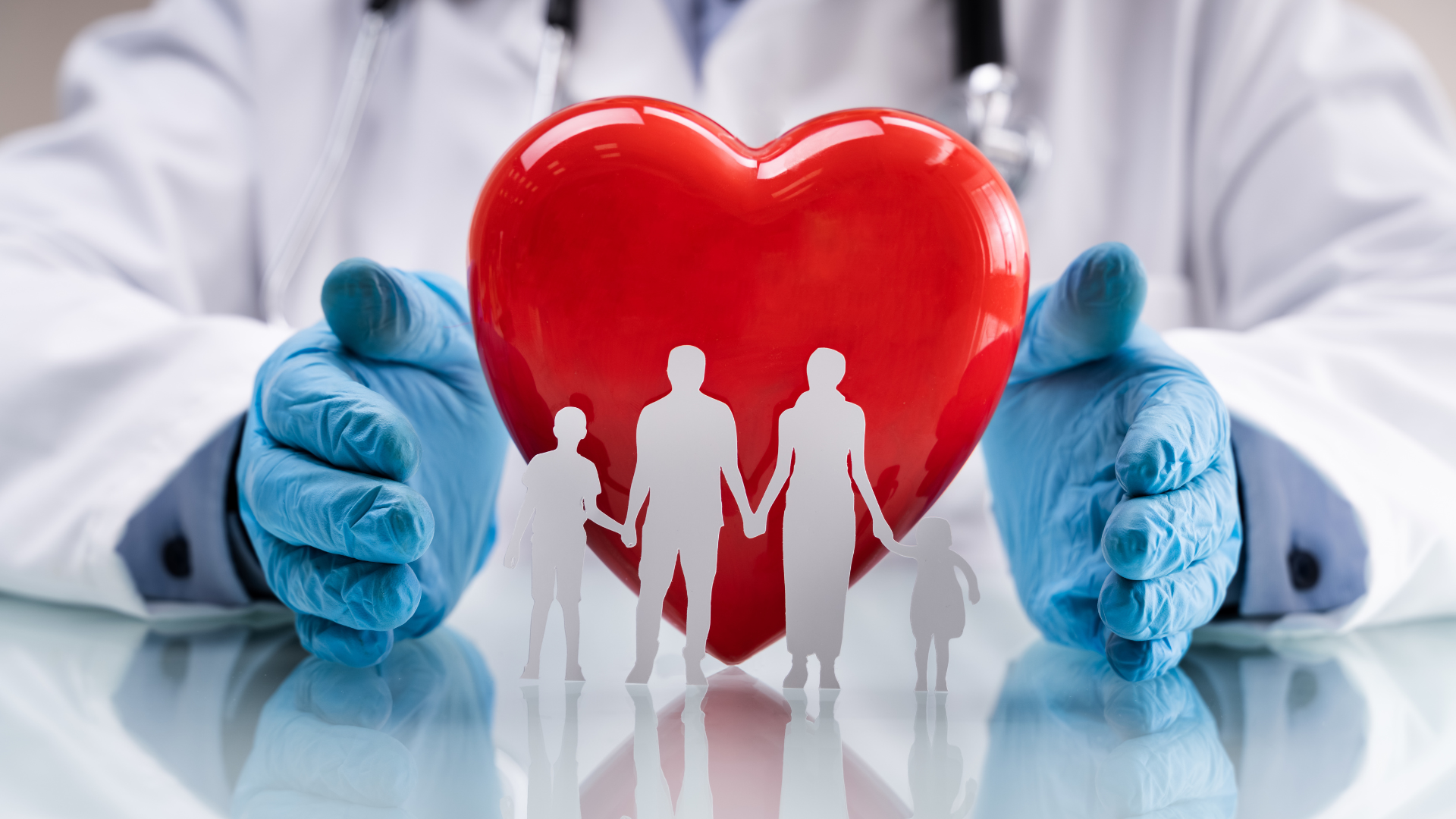